Blackbody-Dominated Gamma-Ray Bursts:
Abstract:
We extend an existing theoretical model to explain the class of blackbody-dominated (BBD) gamma-ray bursts (GRBs), namely long lasting events characterized by the presence of a notable thermal component trailing the GRB prompt emission, and a rather weak traditional afterglow. GRB 101225A, the "Christmas burst", is the most prominent member of this class. It has been suggested that BBD-GRBs could result from the merger of a binary system formed by a neutron star and the Helium core of an evolved, massive star. By means of detailed relativistic hydrodynamic numerical simulations we model the propagation of ultrarelativistic jets through the environments created by such mergers.
In this series of two papers, the output of our numerical models is further postprocessed to obtain the (thermal) radiative signature of the resulting outflow (Paper I). The complete (thermal and non-thermal) output of our models is considered in a companion contribution (Paper II).
In Paper I, we outline the most relevant dynamical details of the jet propagation and connect them to the generation of thermal radiation in GRB events akin to that of the Christmas burst. A comprehensive parameter study of the jet/environment interaction has been performed and synthetic light curves are confronted with the observational data. The thermal emission in our models originates from the interaction between the jet and the Hydrogen envelope ejected during the neutron star / He core merger. We find that the lack of a classical afterglow and the accompanying thermal emission in BBD-GRBs can be explained by the interaction of an ultrarelativistic jet with a toroidally shaped ejecta whose axis coincides with the binary rotation axis.
In Paper II, we focus on explaining the emission properties of the jet evolution computing the whole radiative signature (thermal and non-thermal) of the jet dynamical evolution. The lack of a classical afterglow and the presence of thermal emission in BBD-GRBs are consistent with the interaction of an ultrarelativistic jet with a toroidally shaped ejecta whose axis coincides with the binary system rotation axis. We find that the non-thermal (synchrotron) emission of the forward shock of the jet is dominant during the early phases of the evolution, during which that shock is still moderately relativistic. The contribution of the reverse shock is of the same magnitude than that of the forward shock during the first 80 minutes after the GRB. Later, it quickly fades because the jet/environment interaction chocks the ultrarelativistic jet beam and effectively dumps the reverse shock.
We highlight that, in agreement with observations, we obtain rather flat light curves during the first 2 days after the GRB, and a spectral evolution consistent with the observed reddening of the system. Besides, we obtain that this spectral inversion and reddening happening at about 2 days in the Christmas burst can be related to the time at which the massive shell ejected in an early phase of the common-envelope evolution of the progenitor system is completely ablated by the ultrarelativistic jet.
Movies:
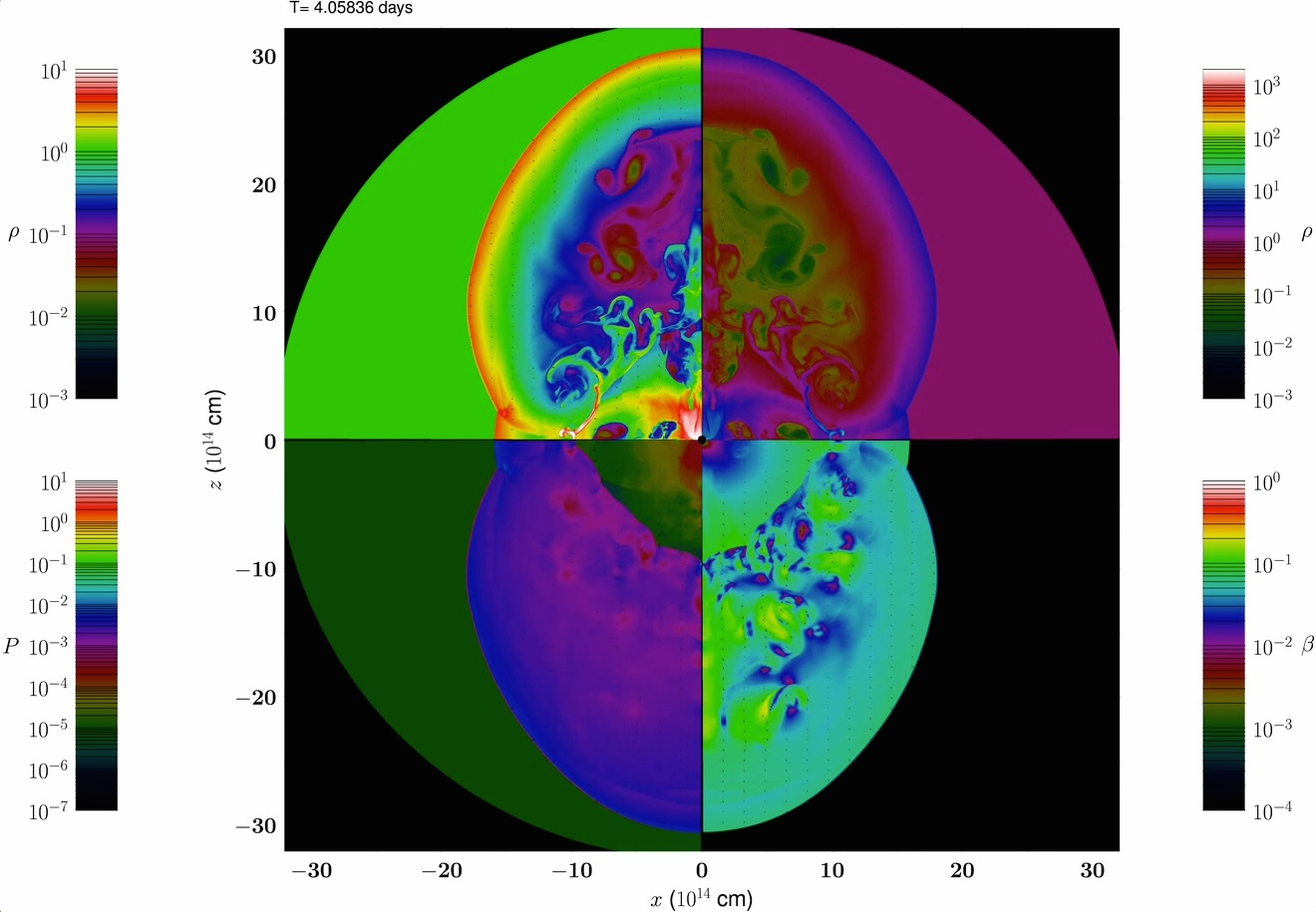 |
Evolution of the Reference Model (RM) up to 4.06 days. We show the rest-mass density in units of rhoRM,ext = 8 · 1014 cm (upper panels), the pressure in units of rhoRM,ext c2 (lower-left panel) and the velocity fluid in units of c (lower-right panel). The CE shell is represented as a massive shell with a toroidal-like geometry. The gap between the injection nozzle and the CE shell is set to let the jet accelerate.
The hydrodynamical evolution can be divided in three phases. In the first one the jet is injected and accelerates (up to ~0.003 days). In the second one, the jet interacts with the CE shell while a small part of it escapes through the funnel (up to ~0.05 days). The jet disrupted qucikyly after the initial interaction. In the third phase the jet material breaks out of the CE shell, expanding sideways almost freely. The interaction of the jet with the CE shell causes the ablation of the latter after ~1 day. At the end of the simulation the expansion of the formed bubble is is quasi-self-similar.
Click here to play the movie RM.avi |
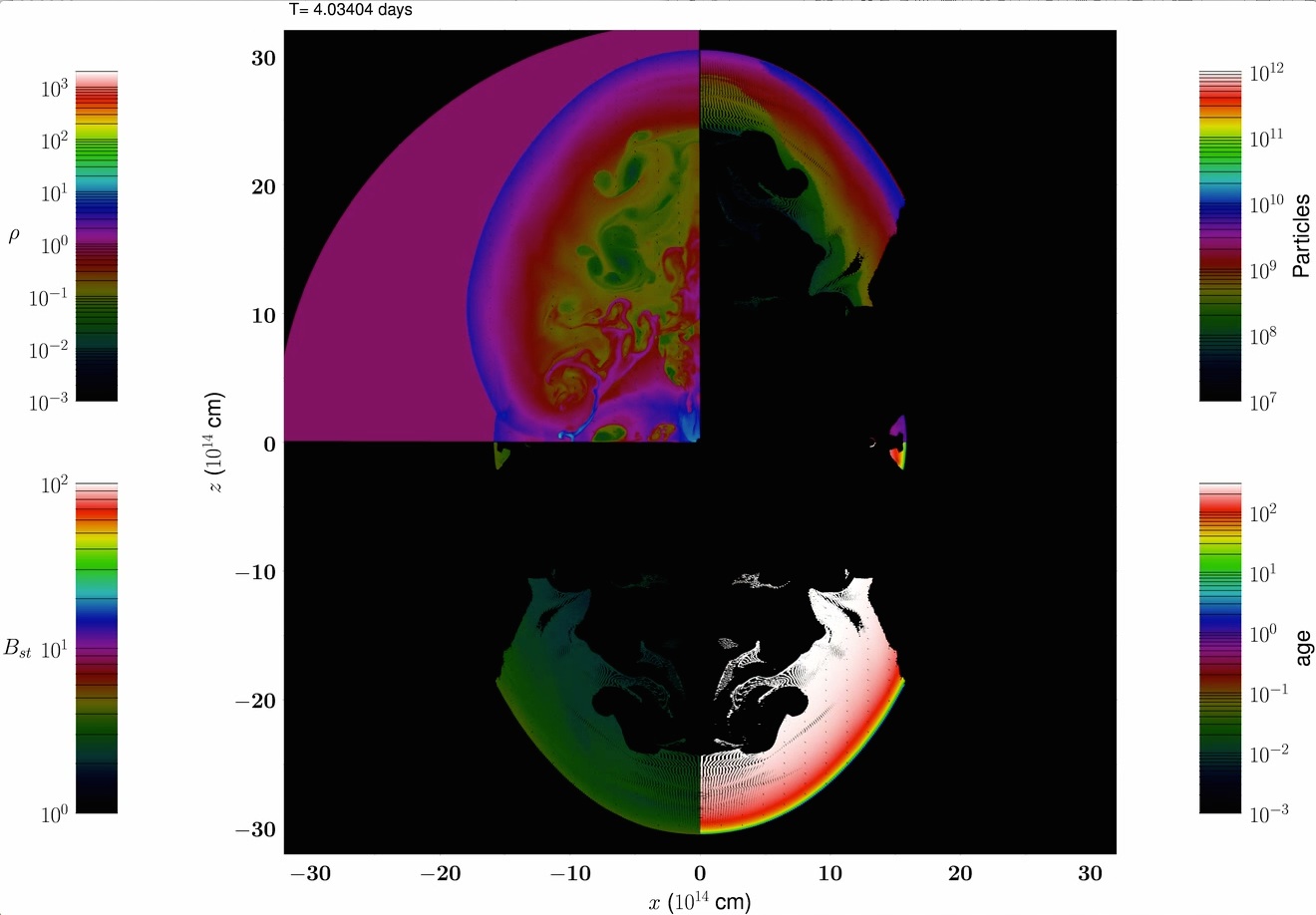 |
Evolution of the non-thermal particles injected in the RM. We show the number of non-thermal particles per cm-3 (upper-right panel) , the stochastic magnetic field in gauss (lower-left panel) and the age of the non-thermal particles in units of R0 / c s (lower-right panel). For comparation we also show the rest-mass density of the thermal fluid in units of rho{RM,ext} (upper-left panel). Only those non-thermal particles which are moving towards the observer (located at theta_obs = 0º) are injected.
Click here to play the movie RM-nonth-part.avi |
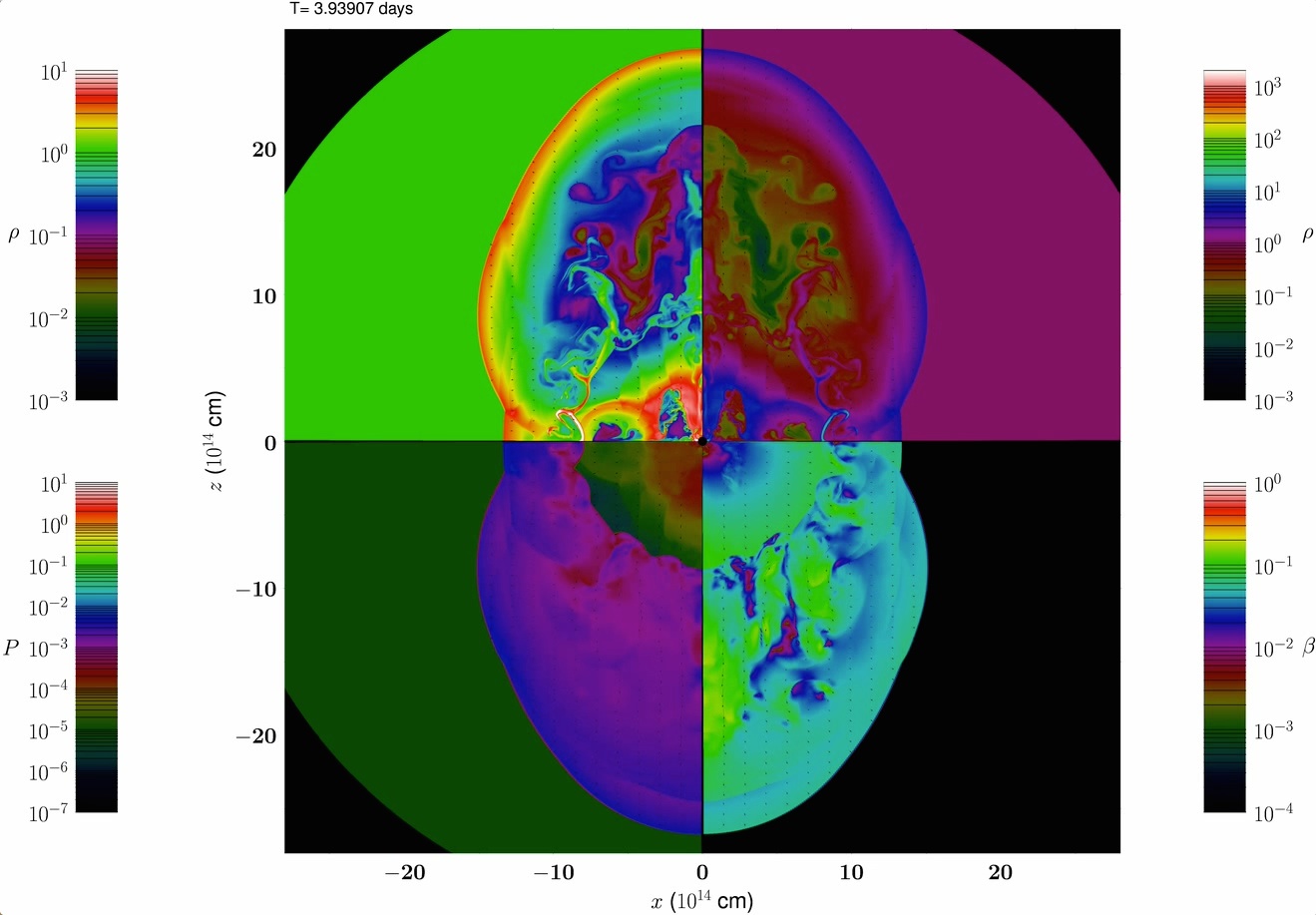 |
Same as RM.avi, but for Model E53. The energy of the jet is 2 times smaller than that of RM.
Click here to play the movie E53.avi |
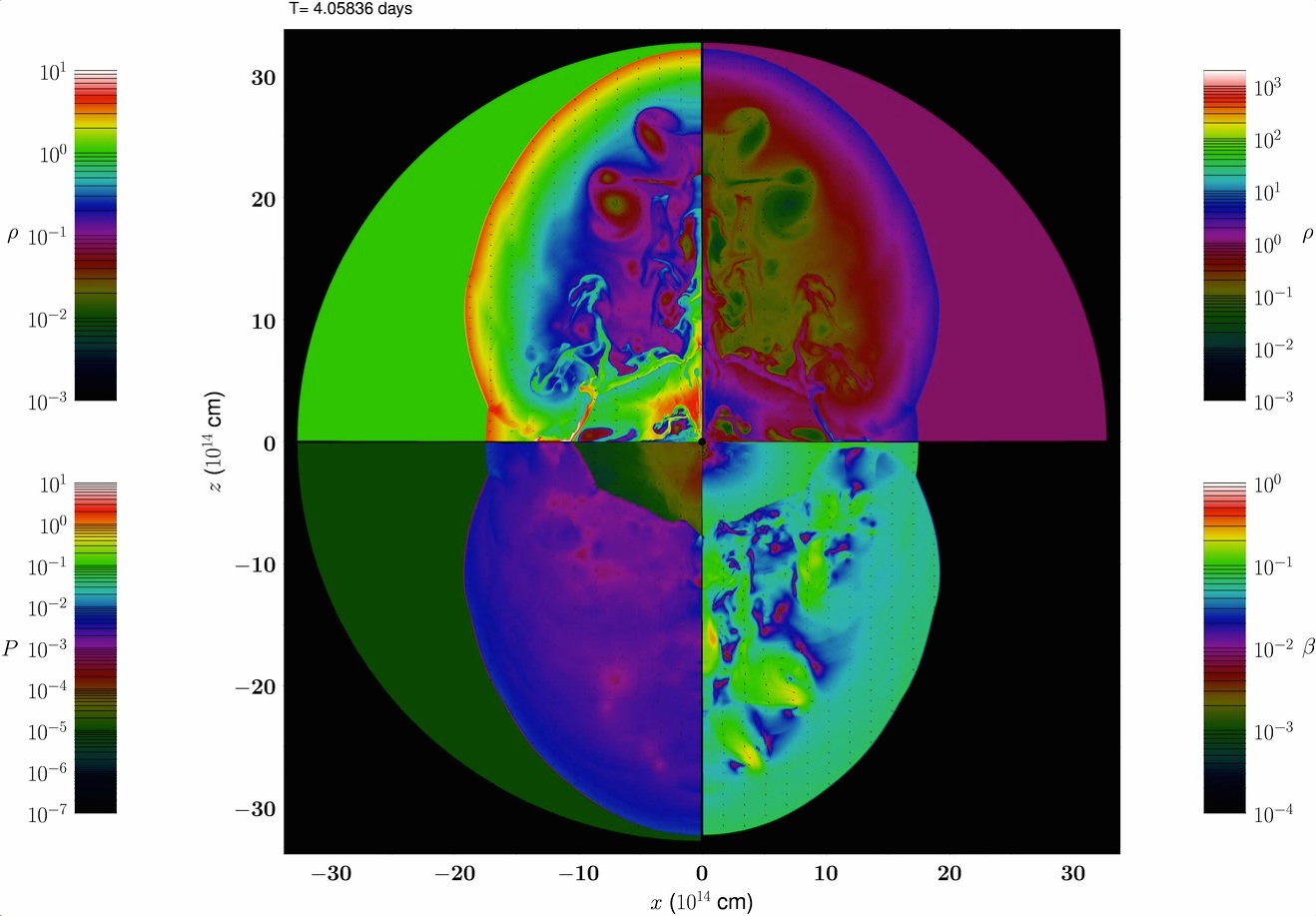 |
Same as RM.avi, but for Model T20. The jet half-opening angle is 20º instead of 17º as in the RM.
Click here to play the movie T20.avi |
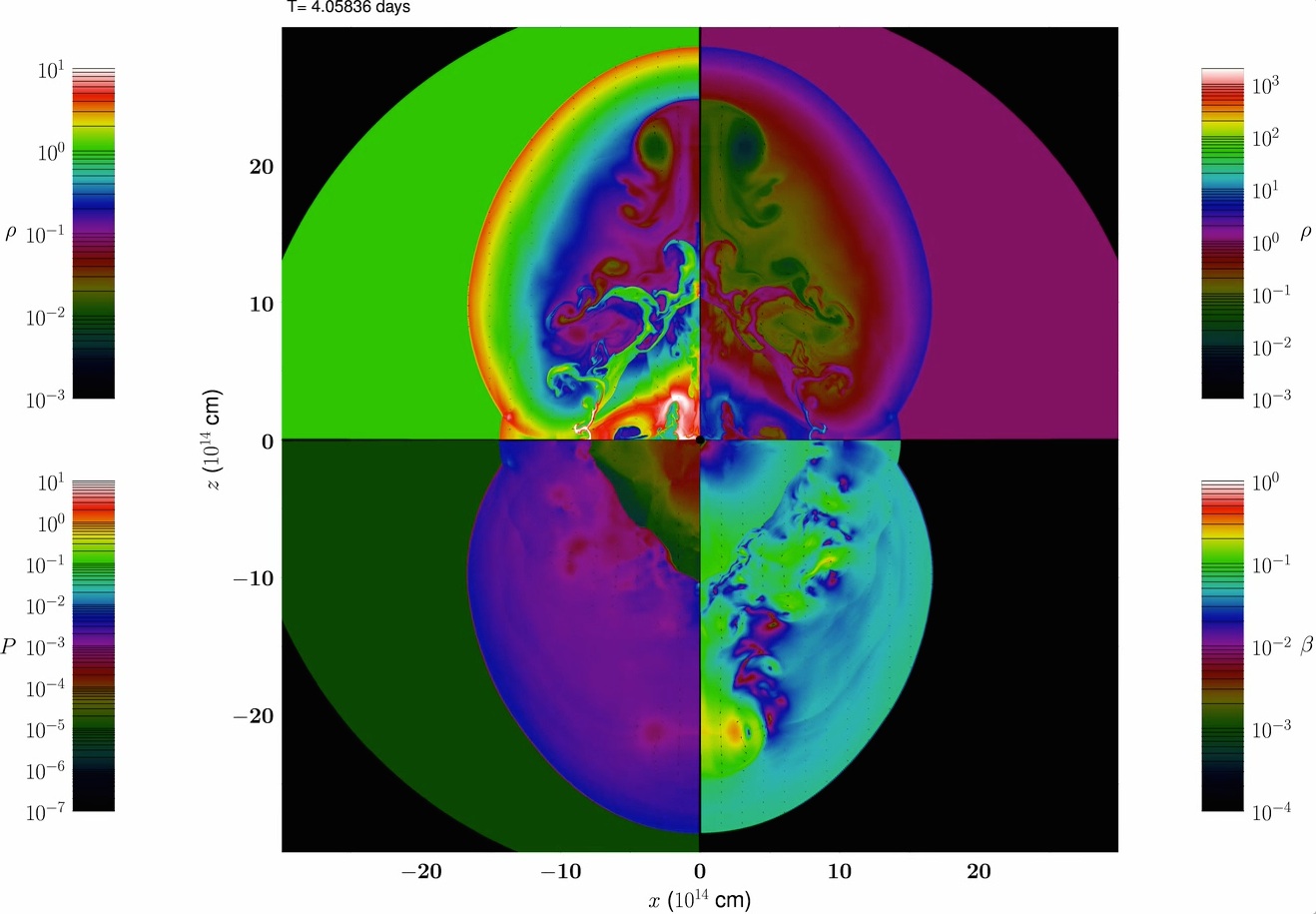 |
Same as RM.avi, but for Model T14. The jet half-opening angle is 14º instead of 17º as in the RM.
Click here to play the movie T14.avi |
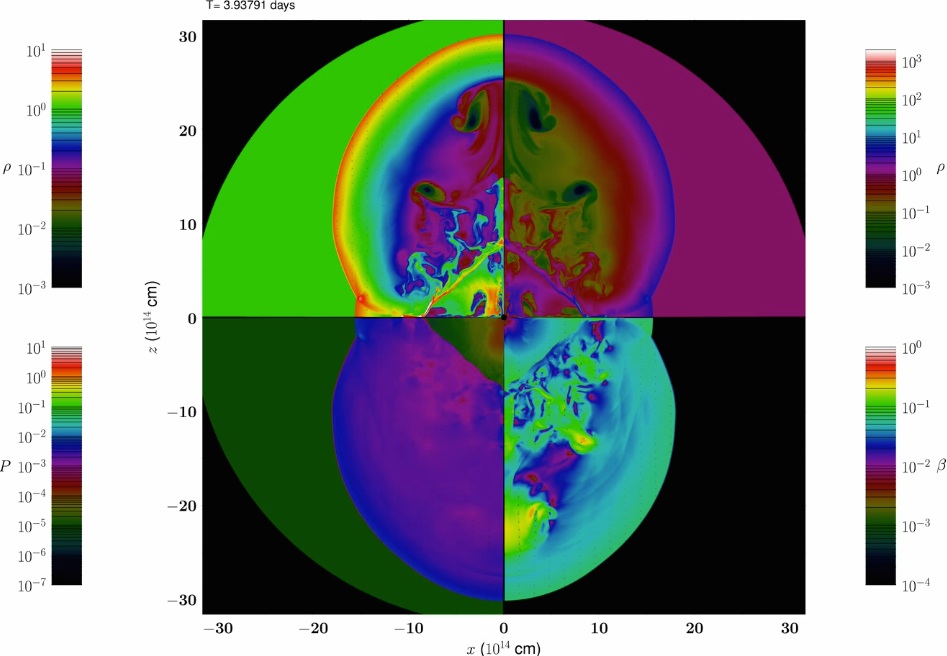 |
Same as RM.avi, but for Model D2. The CE shell rest-mass density is only 817 times larger than the external medium instead of 1500 times larger as in the RM.
Click here to play the movie D2.avi |
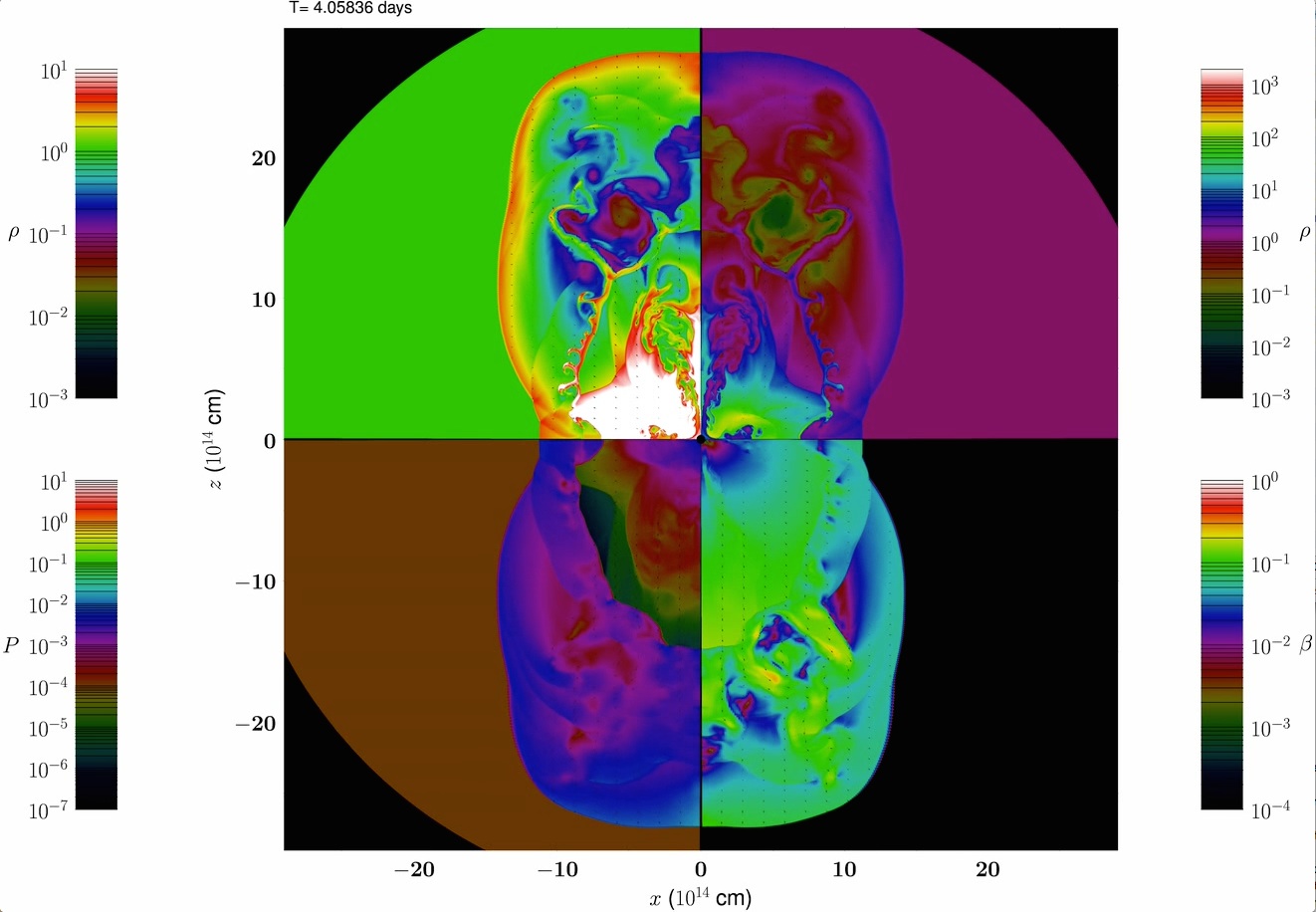 |
Same as RM.avi, but for Model D3. The CE shell is 10 times larger than that of RM.
Click here to play the movie D3.avi |
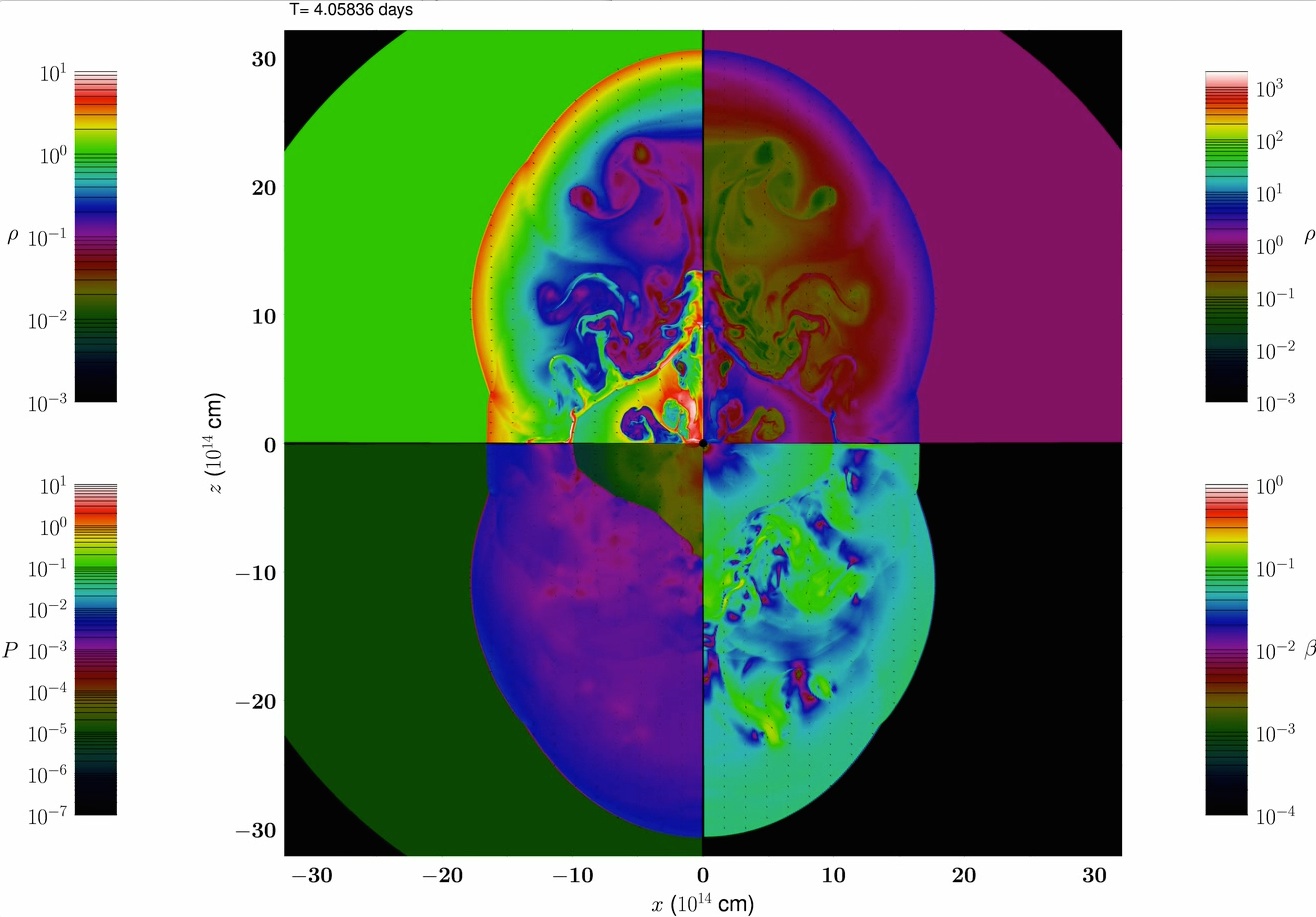 |
Same as RM.mp4, but for Model GS. The CE shell is stratified with a density of 4304 times larger than the external medium in the innermost radius of the CE shell. The total mass of the CE shell remains the same as the RM.
Click here to play the movie GS.avi |
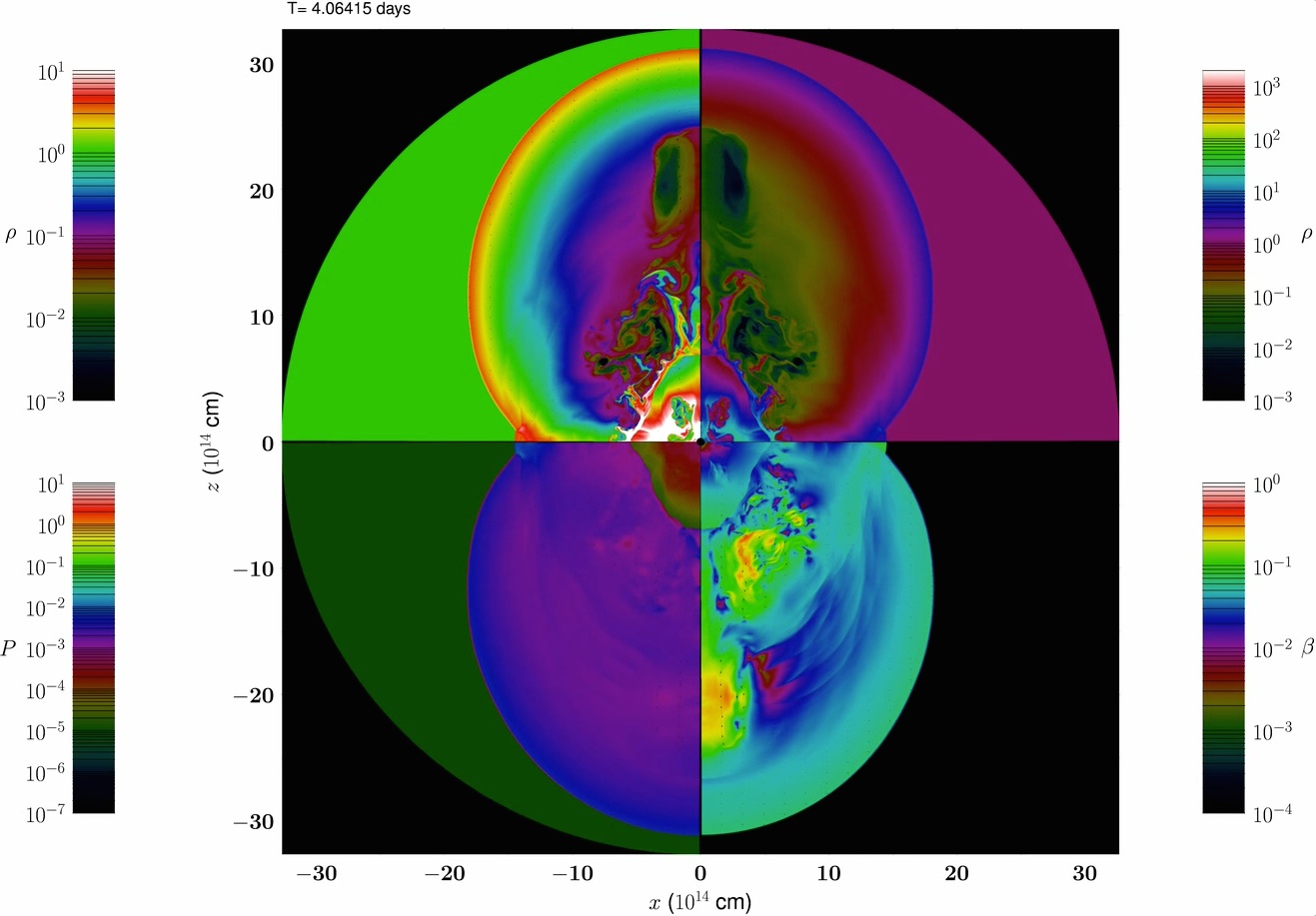 |
Same as RM.avi, but for Model G2. The funnel of the CE shell has a linear geometry.
Click here to play the movie G2.avi |
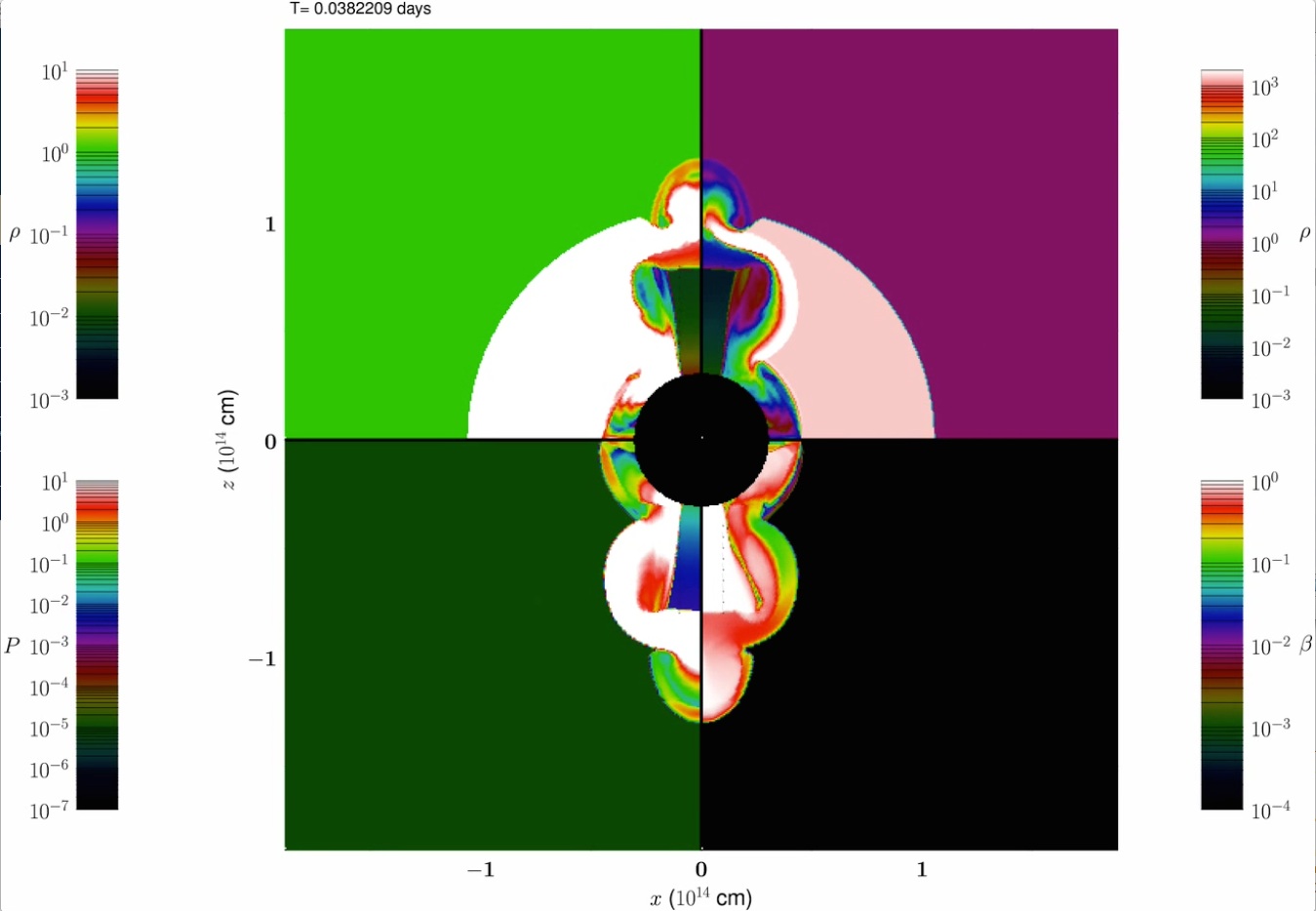 |
Same as RM.avi, but for Model G3. The opening angle of the CE shell is 30º instead of 15º as in the RM.
Click here to play the movie G3.avi |
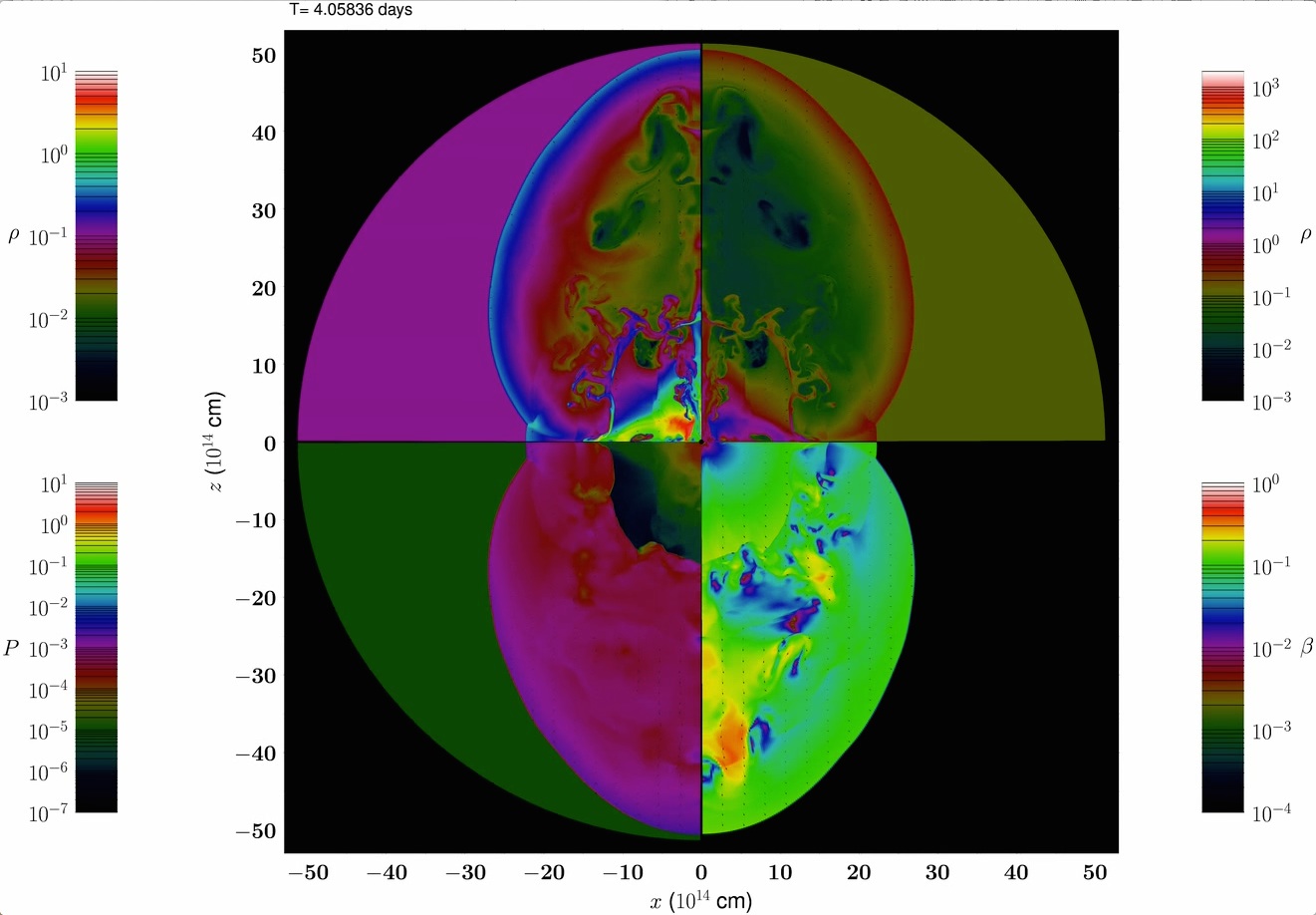 |
Same as RM.avi, but for Model M2. The external medium rest-mass density is 10 times smaller than that of the RM.
Click here to play the movie M2.avi |
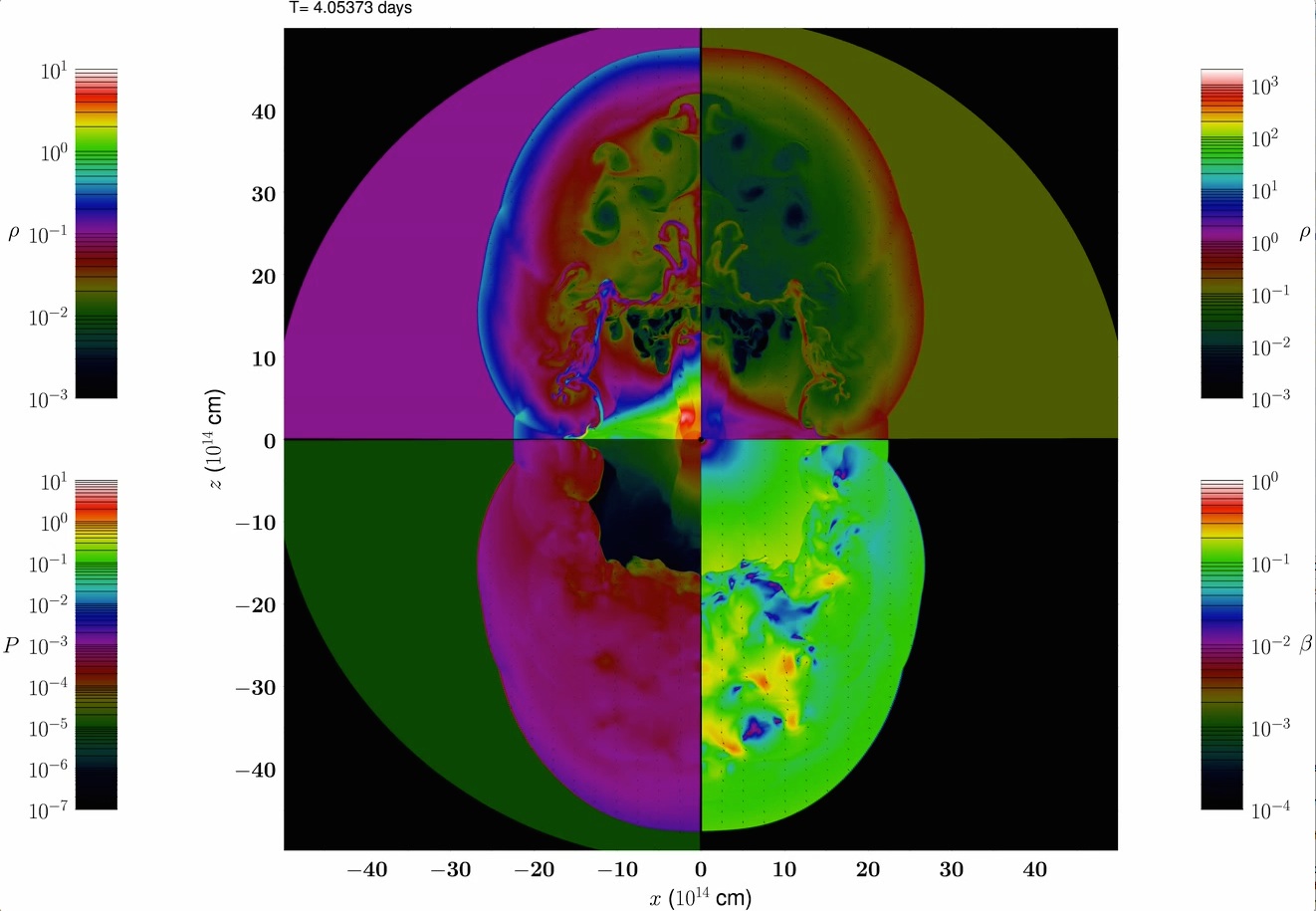 |
Same as RM.mp4, but for Model G0. The external medium has the same properties as in Model M2. Likewise the gap between the CE shell and the injection nozzle has been removed in order to show the small influence of it on the general evolution of the jet.
Click here to play the movie G0.avi |
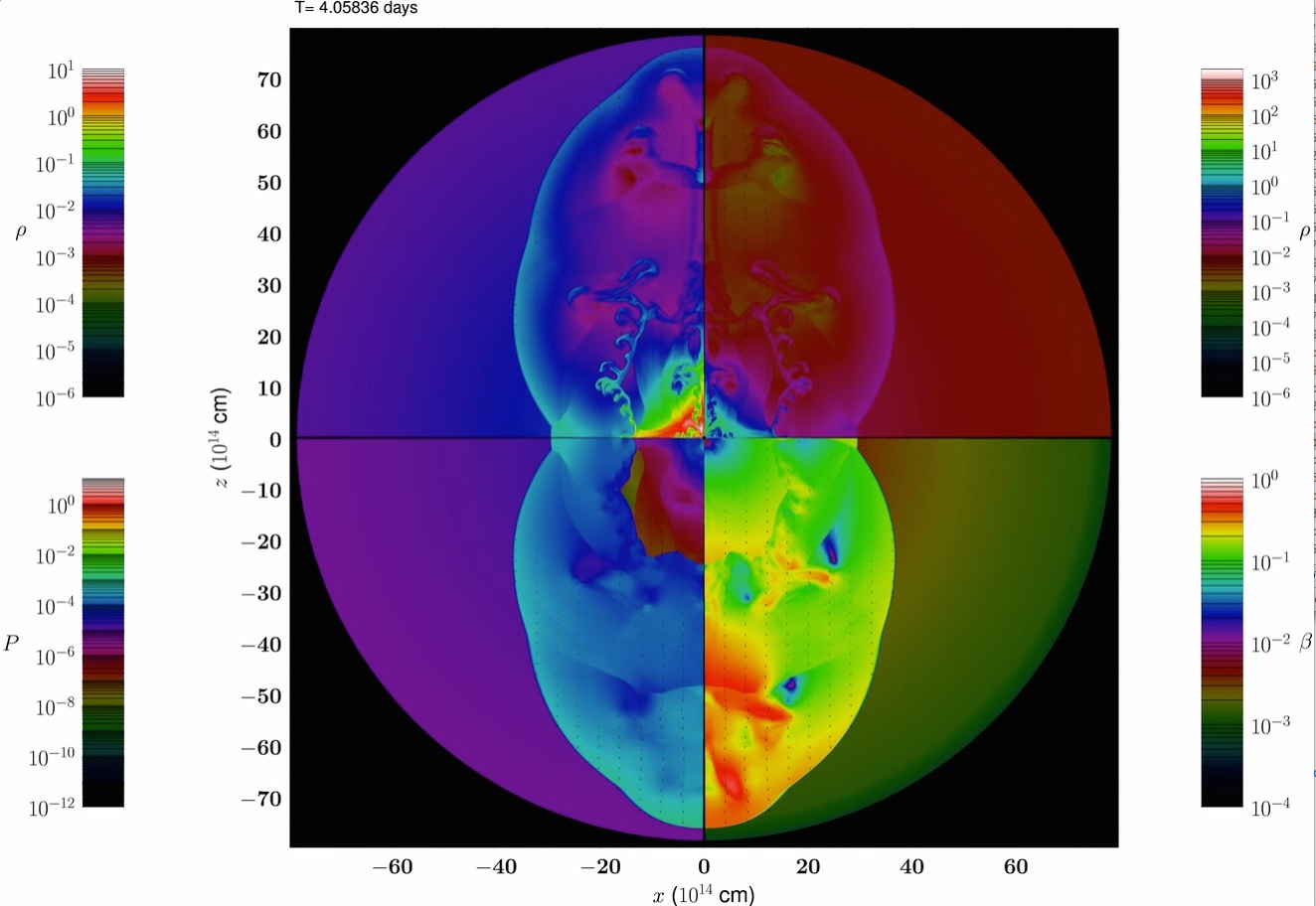 |
Same as RM.avi, but for Model S1. Rest-mass density and pressure of the external medium are stratified as r-1. For convinience the pressure of the external medium in R0 is 100 times larger than that of the RM. Note the change in the colour palette respect to RM.avi.
Click here to play the movie S1.avi |
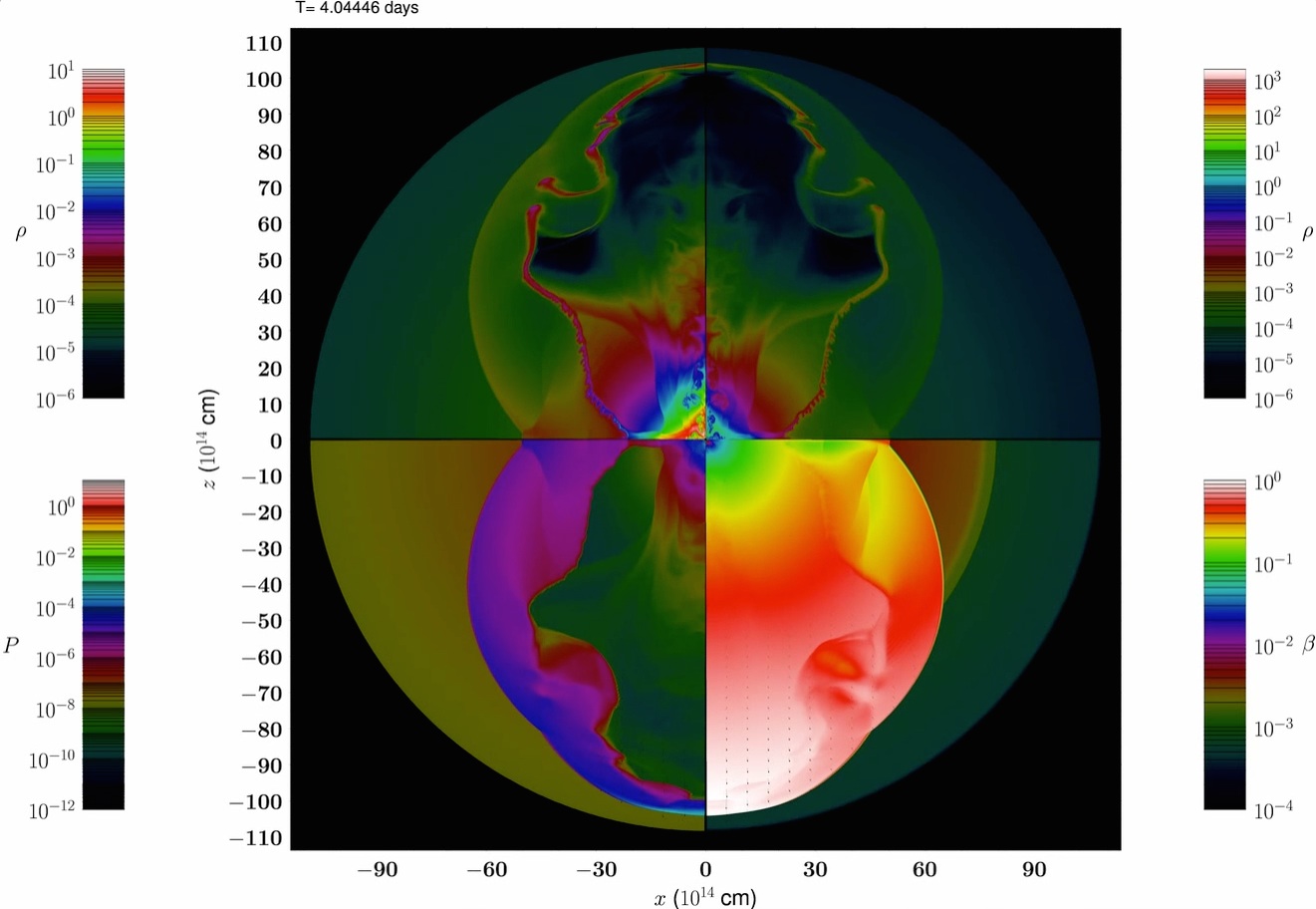 |
Same as RM.avi, but for Model S2. Rest-mass density and pressure of the external medium are stratified as r-2. For convinience the pressure of the external medium in R0 is 100 times larger than that of the RM. Note the change in the colour palette respect to RM.avi.
Click here to play the movie S2.avi |
|