Gravitational Wave Astronomy
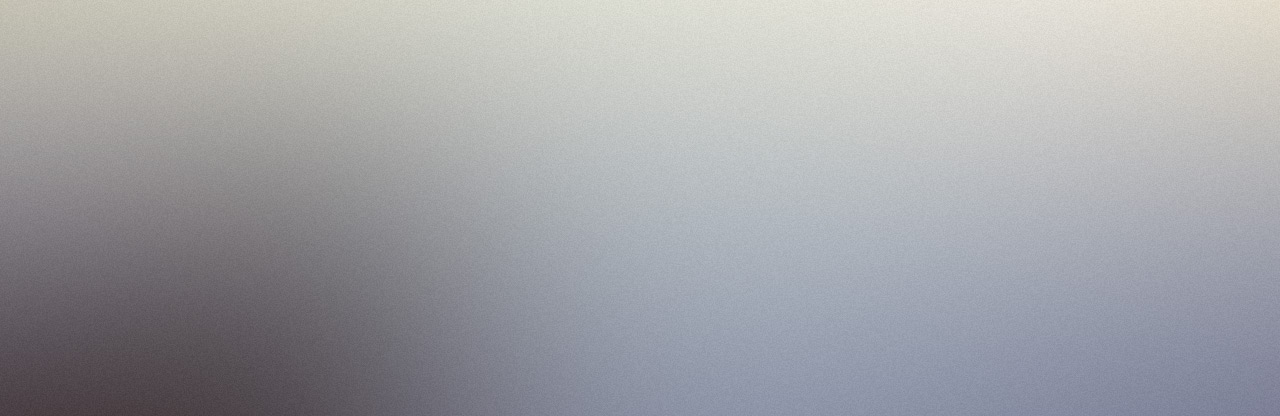
This research line focuses on advancing gravitational-wave (GW) astronomy by contributing to the upcoming fourth (O4) and fifth (O5) observing runs of the LIGO-Virgo-KAGRA (LVK) network, as well as preparing for next-generation detectors such as the Einstein Telescope (ET). The research spans several key areas, including theoretical modeling, numerical simulations, data analysis, and multi-messenger follow-up.
Eccentric Binary Black Hole (BBH) Mergers
The majority of detected BBH mergers exhibit circular orbits, but some binaries formed in dense stellar clusters or galactic nuclei can have measurable eccentricity. The detection of such mergers provides crucial information about their formation channels. With O4 and O5 expected to significantly increase the number of detected eccentric BBH mergers, this project aims to refine waveform models by testing and calibrating the TEOBResumS framework using numerical relativity (NR) simulations. The goal is to improve parameter estimation and waveform templates for future observations.
Binary Neutron Star (BNS) Mergers and Post-Merger Remnants
The landmark detection of GW170817 confirmed that BNS mergers are the progenitors of short gamma-ray bursts (GRBs) and kilonovae, and provided new constraints on the equation of state (EOS) of neutron stars. This project focuses on post-merger physics, particularly the role of magnetic-field amplification and finite-temperature effects in hypermassive neutron stars (HMNS). Advanced numerical simulations will be performed using the Einstein Toolkit, incorporating the newly developed MInIT turbulence model to study angular momentum transport in the HMNS. These studies will improve our understanding of post-merger GW emission and may provide observational signatures for future GW detectors.
Proto-Neutron Star (PNS) Oscillations and Asteroseismology
During core-collapse supernovae (CCSN), the proto-neutron star (PNS) undergoes oscillations that generate GWs in the 10-5000 Hz range. If detected, these signals could provide direct information on the internal structure of neutron stars and the dynamics of supernova explosions. This project builds on previous work by the group, which developed numerical methods to analyze the eigenmodes of oscillating PNSs and established universal relations linking GW frequencies to the mass and radius of the PNS. The project will refine these techniques, extend them to rotating progenitors, and explore the detectability of inertial modes using Bayesian inference.
GWs from Exotic Compact Objects (ECOs)
GW observations provide a unique tool to test alternative theories of gravity and explore new physics beyond the Standard Model. This research investigates the possibility that some observed GW signals originate from exotic compact objects (ECOs) such as boson stars or black holes with bosonic hair, which could provide insights into dark matter. The team will conduct NR simulations of ECO mergers, construct waveform catalogs, and perform parameter estimation on LVK events to determine whether any detected signals deviate from the predictions of classical black hole mergers. A particularly intriguing case study is GW190521, which has been hypothesized to result from the merger of Proca stars.
GW Denoising and Waveform Reconstruction
Given the low signal-to-noise ratios (SNRs) in GW interferometer data, effective noise reduction is critical for reliable detection and parameter estimation. This project enhances GW data analysis by implementing advanced denoising methods based on total-variation regularization and machine learning. These techniques will be integrated into the coherent Wave Burst (cWB) pipeline to improve the detectability of unmodeled burst sources such as CCSN and magnetar flares.
Machine Learning for GW Data Analysis
Deep learning and convolutional neural networks (CNNs) are becoming powerful tools in GW astronomy, particularly for event classification and parameter inference. This project aims to develop machine learning models for detecting CCSN signals and compact binary coalescences (CBC). Previous work by the group has demonstrated that CNN-based approaches can achieve high accuracy in identifying BBH mergers using spectrograms. This research will extend these methods to full CBC searches in existing and future LVK transient catalogs.
Electromagnetic (EM) Follow-Up of GW Sources
Multi-messenger astronomy has become a crucial component of GW science, as demonstrated by the detection of GW170817 and its subsequent EM counterparts across the spectrum. This project continues collaborations with leading EM observatories, including NOEMA (radio), Gran Telescopio Canarias (optical/NIR), and Calar Alto (NIR), to monitor potential EM counterparts to GW events. The team will focus on BNS and NS-BH mergers, as well as potential CCSN GW sources.
Development of New GW Pipelines for O4 and Future Searches
As members of the Virgo Collaboration, the group is actively involved in the development of new GW detection and parameter estimation pipelines for O4. The research will focus on improving the detection of burst sources using machine learning and total-variation regularization methods. The group will also contribute to the development of the TEOBResumS pipeline for eccentric BBH mergers and the pyGRB pipeline for GW-GRB multi-messenger searches.
Participation in Advanced Virgo and Einstein Telescope (ET) Projects
The team plays an important role in Virgo, contributing to data analysis, detector characterization, and outreach. The group participates in service tasks such as monitoring detector performance and responding to candidate GW triggers. Additionally, the team is actively involved in the Einstein Telescope (ET) initiative, co-leading the transient GW division and coordinating outreach efforts. ET is expected to revolutionize GW astronomy by detecting stellar-mass CBCs at redshifts of ∼100 with unprecedented sensitivity.
This research line aligns with LVK priorities and the long-term vision of GW astronomy. By improving theoretical models, enhancing numerical simulations, refining data analysis techniques, and fostering multi-messenger observations, the project aims to maximize the scientific output of the upcoming observing runs. These efforts will contribute to a deeper understanding of compact objects, the physics of extreme environments, and fundamental aspects of gravity and cosmology.