One of the challenges in three-dimensional
microscopy is to overcome the lack of isotropy of the spatial
resolution, which results from the axially-elongated shape of the
point spread function (PSF). Such anisotropy gives rise to images
in which significant axially-oriented structures of the sample are
not resolved. On the other hand, the beam emerging from the
high-NA objective focuses deeply through an interface between two
media of different refractive index. Such a refractive-index
mismatch introduces an important amount of spherical aberration,
which increases dynamically when scanning at increasing depths.
This effect strongly degrades the 3D PSF, and therefore the
instrument performance.
Quasi-isotropic 3-D resolution
The use of wide-field conventional optical
microscopes to image 3D biological fluorescent samples has the
drawback that any image focused at a certain depth in the sample
contains blurred information from the rest of the sample. This
fact gives rise to 3D images with deteriorated contrast and SNR.
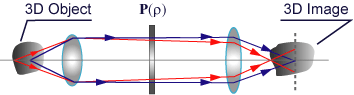
Figure
1.-Schematic
geometry of a wide-field conventional optical microscope
To overcome this problem, the use of scanning
fluorescence techniques has been demonstrated and routinely used.
Among them we cite first single-photon confocal microscopes (SPCM).
The main advantage of SPCM is their sectioning power, due to the
light rejection from out-of-focus parts of the sample.
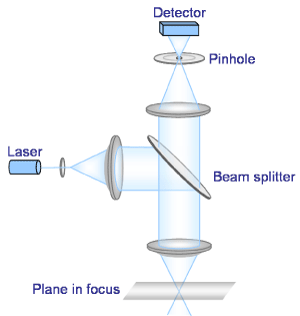
Figure
2.-Schematic geometry of a confocal microscope
Serious drawbacks of SPCM are photo-bleaching,
which occurs since the entire sample is bleached when any single
plane is imaged, and its poor depth-penetration capacity. To solve
these problems, two-photon excitation (TPE) scanning microscopy
was demonstrated. This non-linear imaging technique relies on the
simultaneous absorption of two photons, whereby a single
fluorescence photon is emitted. The overall fluorescent light is
collected and finally the image is synthesized from the 3-D
sampling of the object. TPE generally uses near-infrared light due
to its lower absorption and scatter in biological tissues, which
allows deeper penetration of the excitation beam. As shown in
Fig. 3, in TPE photo-bleaching is restricted to the
neighbourhood of the imaged plane.
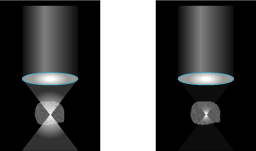
Figure
3.- In TPE fluorescence processes (right-hand figure)
the bleaching is restricted to a small regions surrounding the
focus
We concentrate on apodization of the TPE mode
with the aim of reducing anisotropic imaging, by pupil spatial
filtering with a shaded-ring filter (SR), Fig. 4. We demonstrate that a close to spherical 3-D
PSF can be obtained through the implementation of a relatively
inexpensive spatial filter.
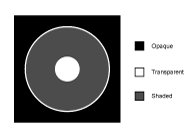
Figure
4.-
The SR filter. The transmittance of the
shaded ring is 19%
In our experiment we used as the illumination
source a mode-locked Cr4+: fosferite laser. The laser
produced pulses with central wavelength
l=1260
nm and a mean temporal width
Dt=50
fs at a repetition rate of 84 MHz. An average power over 200mW was
available for the experiment. We set the coordinate system so that
the incident light is seen as x-polarized. For the illumination
and collection we used two identical 1.2 NA (water-immersion)
objectives from Olympus (Uplan APO/IR 60x). In Fig. 5 we show a scheme of the experimental setup.
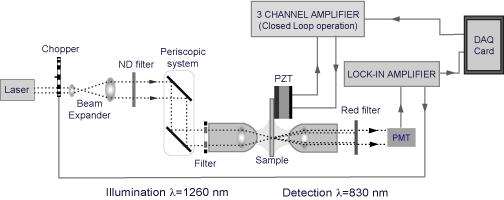
Figure
5.-Schematic
geometry of the TPE scanning microscope
In Fig. 6 we present typical results for the PSF
measurements obtained with and without the SR filter. The
PSF obtained with the SR
is clearly narrowed along the
optical-axis direction, thus exhibiting close to spherical shape.
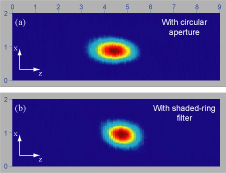
Figure
6.-
2D sections of the experimental 3-D PSFs as displayed by LabView
Quasi-isotropic 3-D resolution can be produced
in TPE scanning microscopy by spatial modulation of the
illumination beam, which involves a small modification of the
microscope architecture.
Reduction of spherical-aberration impact
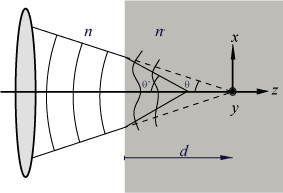
Figure
7.-Focalization of the light through one interface
It is well known that, in a paraxial focusing
regime, an aperture stop with Gaussian transmittance allows the
production of a focused spot whose form changes very slowly with
the spherical aberration (SA). However, a Gaussian pupil is not a
realistic solution for the SA problem in high-NA scanning optical
instruments. This is because, the Gaussian aperture is nothing
more than a reduction of the effective NA of the objective lens.
In Fig.8 we show how the cut-frequency is much bigger in
the case of the circular pupil than for the Gaussian aperture,
both in absence of SA.
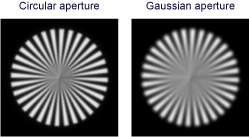
Figure
8.-Numerically evaluated images of a typical spoke
target, in absence of spherical aberration
To take profit from the abilities of Gaussian
transmittances, but avoiding the reductions in effective NA, we
find another pupil, the reversed-Gaussian aperture, with identical
response to SA, but opposite transverse response than the Gaussian
pupil.
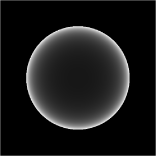
Figure
9. Amplitude transmittance of
the reversed-Gaussian aperture
We have calculated the intensity distribution
in the best focal plane for both cases: the circular and the
reversed-Gaussian aperture. As we see in Fig. 10, in absence of spherical aberration the
circular aperture still produces slightly better results. However,
as the SA increases the response of the circular aperture degrades
very fast, whereas the response of the reversed-Gaussian profile
remains fairly unchanged.
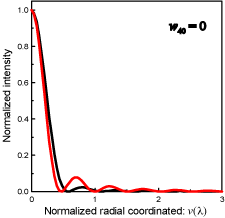
Figure
10.-
Lateral responses corresponding to a high-NA focusing element with
the circular (black line) or the reversed-Gaussian aperture (red
line) as the aperture stop, and for increasing values of w40
To have a visual insight of the improvements
obtained with the reversed-Gaussian aperture we have performed a
numerical imaging experiment. The images were obtained by 2D
convolving the lateral responses in Fig. 10 with the spoke target. The results are shown in
Fig. 11.
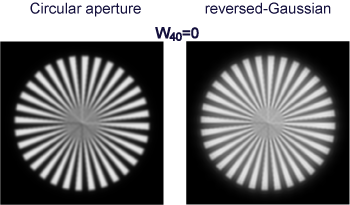
Figure
11.-Numerically
evaluated images of a typical spoke target, for increasing
spherical aberration.
Note that in absence of aberrations the
performance of the circular aperture is slightly better. However,
the reversed-Gaussian aperture is much more robust against the
increasing of the SA. In other words, we can affirm that the
reversed-Gaussian profile confers the high-NA system an important
tolerance to the SA. This tolerance allows the high-NA optical
instrument to have a performance that is not degraded as scans
deeply into a medium of different refractive index.
|